For many who are unable to have a biologically related child due to the absence or quality of their eggs or sperm, newspaper stories about scientists making sperm or eggs from stem cells in the lab may feel game-changing. But what is the science behind these headlines, and what are the limitations in moving forward? This factsheet summarises recent developments towards treating fertility and genetic disease with eggs and sperm made from stem cells.
What do we know?
Stem cells have the ability to grow into different cell types when given the right instructions. Some stem cells can produce any cell type of the body. These are referred to as pluripotent.
Pluripotent stem cells can be derived from five-day-old embryos, before the embryo has implanted into the uterus. These cells (called embryonic stem cells, or ESCs) can be grown in the laboratory in large numbers, while retaining their ability to develop into other cell types.
Pluripotent stem cells can also be created by ‘reprogramming’ other body cells in the laboratory, such as skin cells (these are known as induced pluripotent stem cells, or iPSCs).
These stem cells are of particular interest for use in possible fertility treatments, as scientists might be able to be use pluripotent stem cells to make sperm and eggs (collectively called gametes) for in vitro fertilisation. The process of creating sperm and eggs in the lab is called in vitro gametogenesis, or IVG.
While mice have been made using eggs and sperm generated from stem cells and IVG, this has not yet been achieved in humans.
What are researchers working on?
Ongoing research is focusing on different aspects of in vitro gametogenesis:
- Some groups are pioneering new methods to make egg and sperm precursor cells (primordial germ cells, or PGCs) from human pluripotent stem cells, and then working out how to direct these to develop into sperm or eggs.
- Other groups are trying to create a supportive environment in which these precursor cells can develop into sperm or eggs in the laboratory, which means recreating the developing testis or ovary. This involves directing stem cells to become other cell types of the testis (such as Sertoli cells, which support PGC development, or Leydig cells, which make testosterone) or of the ovary (like granulosa cells, which support egg development).
- These methods are also being used to understand conditions where testicular or ovarian development is disrupted, which in turn affects fertility or sex characteristics. This type of research is called disease modelling. It can include exploring what genes are needed for different steps in making functioning ovaries and testes, as well as eggs and sperm.
What are the challenges?
If eggs and sperm could be made from other adult body cell types through IVG, it could have extensive applications for individuals affected by infertility or the possibility of passing on genetic diseases. However, there are currently four major scientific barriers to applying IVG technologies in humans:
- Making sufficient numbers of viable egg and sperm precursor cells (PGCs) from stem cells.
- Making sure these human PGCs make eggs and sperm, but do not generate tumours, which can be a risk of pluripotent cells.
- Working out how to grow human PGCs into fully functional eggs and sperm cells in the lab, to avoid transplanting immature PGCs into people. This would eliminate invasive procedures and the risk of tumour formation.
- Establishing how to test the ability of laboratory-generated sperm and eggs to create healthy embryos and children, given current restrictions in many countries on creating embryos for research or growing these in the laboratory beyond certain developmental timepoints.
Alongside overcoming the scientific barriers, next steps must also include consideration of the significant social, ethical and legal barriers to the use of IVG. Legislation guiding the use and creation of embryos varies around the world. If IVG technologies become scientifically feasible, legislation will need to be amended to allow for clinical application.
Introduction to Assistive Reproductive Technologies
Assistive reproductive technologies (ART) are technologies which support couples or individuals to achieve a pregnancy without sexual intercourse. This involves manipulation of sperm, eggs or embryos outside the body. ART procedures include the use of in vitro fertilisation (IVF), where embryos are created in the laboratory before being transferred to the uterus of an intending mother or surrogate. ART may also involve the use of donated gametes (sperm or eggs) or fertilised embryos.
ARTs may be used for a variety of reasons:
- Infertility: The World Health Organisation reports that one in six adults experiences infertility, and may therefore be unable to conceive. Infertility can be caused by a range of conditions and factors, and can affect any individual.
- Use of donor gametes: Individuals experiencing infertility, same-sex couples, or individuals who wish to conceive and parent singly may choose to conceive using gametes donated by someone else.
- Known genetic risks: If one or both parents know that they are a carrier for a life-limiting genetic condition, they may choose to conceive by IVF and undergo pre-implantation genetic testing. This process allows the embryos to be screened, selecting the embryos that do not carry the genetic mutation for implantation to prevent the parent’s specific genetic condition from being passed on to the child.
Current treaments and support
For individuals or couples with low fertility or who cannot conceive, ARTs, including in vitro fertilisation (IVF) allows the fertilisation of eggs with sperm in the laboratory. Mature eggs are collected from the ovaries; this involves medicines which suppress the natural menstrual cycle and combinations of hormones to help stimulate the maturation of egg cells. Eggs are then collected and fertilised with sperm in the lab.
The resulting embryos are grown for up to 6 days and their development is assessed. At this point, if either parent is a carrier for a life-limiting genetic condition, they may choose pre-implantation genetic testing, which allows the embryo’s genes to be screened in the laboratory. Embryos without the mutation which causes the condition are identified, so that the condition is not passed on to the child. Embryos can then be transferred into the uterus of the intending mother or surrogate, or frozen and stored for future use.
However, for current ART technologies to result in a biologically related embryo, at least one parent is required to have viable gametes.
For individuals and couples who lack suitable sperm or eggs, donor gametes or donor embryos may be offered. This would mean the resulting child will not be genetically related to one or both intending parents. Accessing donor gametes or donated embryos can be challenging due to the limitations and restrictions around donation, and the limited number of donors.
Currently, additional non-ART options are adoption and the choice to remain childless.
How might gene and cell therapies help?
To create a biologically related embryo, existing ARTs require that at least one parent has healthy, functional egg or sperm cells. However, researchers are investigating how to produce healthy eggs and sperm from stem cells in the laboratory (called in vitro gametogenesis, or IVG), but this has not yet been achieved in humans.
There are several reasons why an individual or couple could potentially benefit from IVG:
- Existing ARTs have been unsuccessful.
- Existing ARTs are not suitable due to lack of ovaries/testes eggs/sperm, or infertility as a result of age or cancer treatment.
- Couples are known carriers of genetic conditions and wish to create a larger pool of embryos to select from.
- Avoiding the risks and burdens of existing ARTs, which may include hormonal injections, close monitoring and egg collection procedures.
- Same-sex couples who only naturally produce either eggs or sperm.
- Single individuals who are infertile and wish to create an egg or sperm from their own stem cells to be fertilised with a donor.
- Single individuals who wish to use their own natural egg or sperm with a corresponding stem-cell derived egg or sperm from themselves, allowing them to be the sole genetic parent.
What are pluripotent stem cells?
Pluripotent stem cells can become any other cell type in the body, from muscle, nerve, skin cells to eggs and sperm. While an embryo is developing, populations of stem cells will mature into each different cell type, losing their pluripotency. However, there are methods for ‘reprogramming’ a mature cell back to this pluripotent state, and these 'induced' pluripotent cells (iPSCs) can then produce any type of body cell once again.
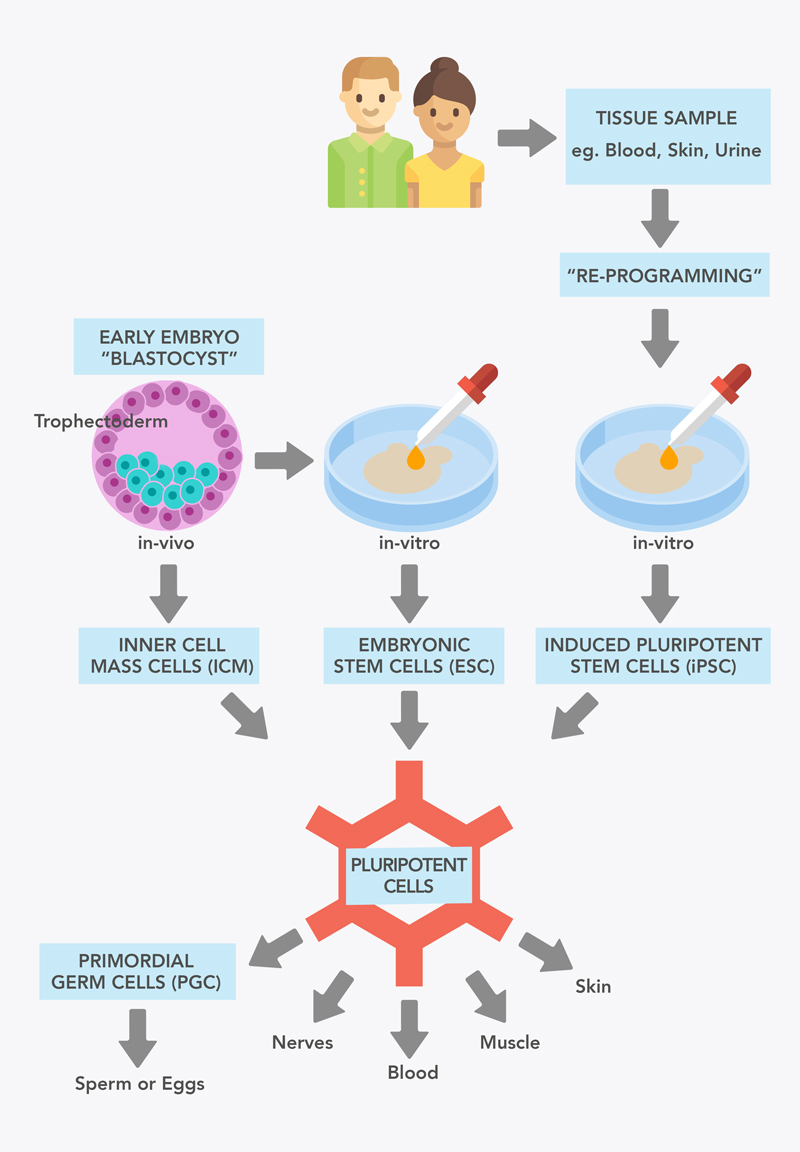
Figure 1: Sources of pluripotent stem cells.
A type of pluripotent stem cell can be obtained from a very early stage of embryonic development (see Figure 1). At five days, the embryo is called a blastocyst. This consists of an outer layer of cells called the trophoblast, and an inner layer called the Inner Cell Mass (ICM). The cells of the ICM are pluripotent, but after the embryo implants in the uterus, these cells go on to develop into the embryonic cells and tissues.
When ICM pluripotent cells are grown outside the embryo in the lab, they are known as embryonic stem cells (ESC). ESC were the first population of pluripotent stem cells (PSC) available to researchers, first derived from human embryos in 1998.
There are no well-established sources of pluripotent stem cells in children or adults, as most of these have already matured and are committed to becoming a specific type of cell. However, in 2006, the researcher Shinya Yamanaka showed that Shinya Yamanaka showed mouse skin cells could also be made into stem cells. This process is known as ‘re-programming’. These cells resembled embryonic stem cells, and were named induced pluripotent stem cells (iPSCs). These cells, like embryonic stem cells, have the potential to form all cell types of the body, including potentially eggs and sperm. This technology was soon shown to work for human cells too. The generation of iPSCs was revolutionary to the stem cell field and medicine as a whole, and was awarded the Nobel Prize in 2012 only 5 years later. iPSCs have now been made from many cell types including blood cells, hair follicle cells, and even cells collected from urine!
Using pluripotent stem cells to make healthy sperm and eggs
In mice, pluripotent stem cells have been used to make sperm and eggs from mouse cells. Eggs and sperm have not been made from human pluripotent stem cells, although there has been some success making the precursor cells (primordial germ cells, PGCs)
Researchers from Japan, Katsuhiko Hayashi and Mitinori Saitou, pioneered in vitro gametogenesis (IVG) technology using animal models. In 2011, they showed that PSCs from mice could be reprogrammed to become sperm, which in turn could develop to healthy and fertile pups. The following year, they showed that female mouse PSCs could be turned into eggs that could also develop to healthy and fertile pups.
Several factors were critical to this success:
- The starting cell type: Following embryo implantation the Inner Cell Mass turns into ‘epiblast’ cells. Some of these epiblast cells exit the developing embryo and turn into primordial germ cells (PGCs). These PGCs later migrate back into the foetus and take residence in the forming ovaries or testes, where they turn into eggs or sperm respectively. Some researchers have investigated how to produce cells similar to these epiblast cells. However, Hayashi and colleagues showed that these cells were not very effective at making PGCs. Instead, they developed a new type of epiblast cell from mouse ESCs: the Epiblast-like cell. This cell type is more successful at turning into PGCs, and subsequently, eggs and sperm.
- Cell type purity: When turning pluripotent stem cells into specific types of cells, it’s difficult to get every cell to respond in exactly the same way. This can lead to a mixed population of cells, including types you don’t necessarily want or cells that retain pluripotency and fail to form eggs and sperm. These cells can also form tumours. Purification of PGCs is critical for removing those unwanted cell types, and these studies developed ways to do this.
- A requirement for in-vivo maturation: The process of making functional, mature eggs and sperm in these studies required transplantation of the PGC-like cells back into mouse ovaries or testicles respectively. Here, they were supported to develop further into eggs and sperm, something that was not at the time achieved in the laboratory. Finding out how the ovary/testicle supports this maturation is important: it will allow researchers to try to recreate these conditions in the lab, removing the need for invasive and risky procedures or functional testis and ovaries.
Further research has continued to improve this technology. In 2016, Saitou and Hayashi showed that mouse ESCs and iPSCs could be developed into mature eggs in a dish, without the need to put cells back into mouse ovaries for maturation. However, they still required dissected tissue from mouse ovaries to support the development of the eggs.
The requirement for mouse ovarian tissue has recently been overcome by work from Hayashi’s laboratory, when ovarian supporting cells were created from stem cells. These cells were able to support the development of stem cell-derived PGC-like cells into viable eggs, that could be fertilized and produce viable embryos.
Similarly, work from the Zhou lab in China showed the creation of sperm precursor-like cells from mouse ESCs without the requirement for testis tissue, allowing full IVG without the need for tissue from an animal model for the first time.
What about in humans?
Some researchers have tried to recreate these findings using human cells, but so far, they have not been able to make functional sperm or eggs from human stem cells. There are still gaps in our understanding of natural human embryonic development. Since the first success in making PGC-like cells from human stem cells in vitro, a number of research groups have developed more efficient or refined methods to produce the gamete precursors. However, maturing these into functional eggs and sperm remains a challenge.
In 2018, Yamashiro and colleagues created cells that resemble early human egg cells from iPSCs. However, they relied on the use of mouse ovarian tissue to support the differentiation of the cells. Similarly, in 2021, Hwang and colleagues derived human sperm precursor cells from iPSCs, but again relied on mouse testicular cells to support development.
Pre-implantation Genetic Testing
Individuals or couples who know that they are carriers for a genetic condition may wish to avoid passing this condition to their child. There is currently only one way to actively avoid implantation of an embryo carrying the genetic disease; Pre-Implantation Genetic Testing (PGT), which can only be used alongside IVF.
After embryos are fertilised in the clinic for IVF, they are cultured for several days until they reach the blastocyst stage of development, consisting of the inner cell mass and surrounding trophectoderm. The trophectoderm are the cells that go on to form support structures such as the placenta and amniotic membranes during pregnancy. At this stage, parents may opt for PGT.
4-5 cells are removed from the trophectoderm. These cells are genetically sequenced for the disease variant. Embryos which do not carry the genetic variant for the condition are identified and can be transferred to the uterus of the intending mother or surrogate, or frozen for future use. This reduces the number of embryos a couple can use, and can therefore require a larger number of starting embryos. In some cases, multiple rounds of IVF are needed before a pregnancy with an embryo not carrying the genetic variants can be achieved. It is not possible to genetically test eggs and sperm prior to fertilisation, as analysing the cell’s DNA with current techniques would destroy the cell.
It has been proposed that gene editing could potentially be used to correct mutations in screened embryos, instead of selecting those without mutations. However, there are significant technical and ethical considerations that prevent this. Current gene editing technology may affect non-targeted parts of the DNA, causing unwanted side effects. It would also be difficult to correct the mutation in every cell of the embryo, resulting in some cells carrying the mutation and potentially affecting the health of the child.
Producing eggs and sperm from pluripotent stem cells could provide an alternative to attempting to edit the genes in an embryo. This is because the genetic variant could be corrected in the stem cells before they are used to make the gametes. Correcting variants in stem cells is already a well-established technology. The cells can then be screened for off-target mutations, before eggs or sperm are made. In addition, because stem cells can be easily grown in large numbers, a larger number of gametes could be produced than would typically be collected in an IVF cycle. Below are some examples of how inheritance of genetic diseases could be avoided.
CRISPR/Cas9 genome editing: The DNA code of stem cells could be altered. This could mean adding a ‘missing’ section, removing a section which codes for a disease gene, or correcting a genetic variant in a gene so that it codes for a healthy gene. Corrected cells would then be selected and grown. This would mean that all resulting eggs and sperm made from these corrected stem cells would not carry the disease-causing variant. Unintended mutations (off-targets) could also be tested for prior to production of eggs and sperm.
- Selection: For conditions caused by mosaicism (conditions where a genetic change is found in only some cells), single pluripotent stem cells not carrying the disease-causing variation could be identified and isolated. These cells could then be grown for egg and sperm production.
- Removal of extra chromosomes: some conditions that affect fertility are caused by chromosomal aneuploidy, where an individual carries too many or too few chromosomes. In this case, stem cells could also be used to make gametes without the aneuploidy. In mice, scientists have successfully removed extra chromosomes from pluripotent cells and used them to make healthy pups. Others have also altered the chromosome number for human iPSC cells models for Down Syndrome (Trisomy 21, where an extra chromosome 21 causes a number of issues including infertility) and Klinefelter Syndrome (where individuals have XXY chromosomes). This could be applied to other human disorders caused by the presence of extra chromosomes. Similar technology could potentially be used for individuals who produce eggs or sperm with sporadic addition or removal of chromosomes, a phenomenon that increases with ageing.
Next Steps
Currently, there are four major barriers to overcome before we can apply the process used in the mice studies to humans:
In 2015, a British human study succeeded in making PGCs with quite high efficiency, with no supporting tissues; around 40% of the cells developed into PGC-like cells. They also believe they have found a way to purify this human PGC-like population to avoid tumours. This study makes strides towards resolving barriers 1 and 2. However, no efforts to mature or transplant these cells were reported.
Other laboratories are putting efforts into creating the supporting somatic cells of the testis or ovaries, and even creating 3D in vitro replicates of ovary or testis tissue (referred to as organoids) derived entirely from stem cells. This may be required to produce fully functional gametes in vitro, and could be also used for many other reproductive medicine applications such as drug screening, disease modelling and assessing the impact of environmental pollutants.
Addressing legal, ethical and social challenges
Alongside overcoming the scientific barriers, next steps must also include consideration of the significant social, ethical and legal barriers. Legislation guiding the use and creation of embryos varies around the world, and often reflects the unique culture and social standards in each country, as well as existing legislation for similar areas of research and medicine. If IVG technologies become scientifically feasible, legislation will need to be amended to allow for clinical application. Any regulatory reform is likely to be influenced by community views on IVG and reproductive technologies, and specifically how key ethical considerations will be addressed such as who should be eligible to use these technologies, how equitable access to the technologies will be ensured, and importantly, what supports will be put in place to appropriately monitor the health of any resulting children over time.
Could we make eggs from male stem cells, or sperm from female stem cells, in a dish?
There is currently no evidence in humans that IVG could be used to create sperm from female stem cells or eggs from male stem cells. However, this has recently been achieved in mice.
What evidence do we have for or against this being possible?
Evidence for production of eggs from XY (male) pluripotent stem cells or sperm from XX (female) pluripotent stem cells:
In 2018, Li and colleagues created live mice with two mothers (bimaternal), and live mice with two fathers (bipaternal). However, the bipaternal mice were not viable long-term as they died shortly after birth.
In 2023, Murakami and colleagues successfully converted XY (male) stem cells to XX (female) stem cells. These XY --> XX stem cells then went on to produce mature and functional eggs at the same efficiency as natural XX stem cells. When fertilised, these eggs went on to produce live offspring, therefore providing evidence of bipaternal reproduction in mice.
Evidence against production of eggs from XY (male) cells or sperm from XX (female) cells:
While the 2023 paper by Murakami and colleagues indicate that this might be possible, decades of previous research into mammalian gamete formation and sex selection questioned whether it is possible to form eggs from cells with XY chromosomes, or sperm from cells without them (reviewed in Taketo 2015). These previous studies argue that production of sperm from XX (female) cells or eggs from XY (male) cells was not possible. Additionally, the barrier to the development of healthy individuals is seen in human sex chromosome aneuploidies associated with sterility including;
- XY females - Swyer syndrome
- XX males - de la Chapelle syndrome
- XXY males - Klinefelter syndrome
- XO females - Turner syndrome
It is important to note that most of these studies look at conditions where both the eggs or sperm and the supporting environment of the ovaries or testes carry dysfunctional genetics. Experiments which put healthy XY PGCs into a healthy XX ovary, or healthy XX PGCs into a healthy XY teste, have not yet been performed. However, these experiments would be theoretically possible using the Hayashi methods discussed above.
More research is required to see if the work of Murakami and colleagues can be reliably repeated.