Lung stem cells in health, repair and disease
Lung diseases – including chronic obstructive pulmonary disease (COPD), cancer, lung fibrosis and pneumonia – are among the most common diseases in Europe. Chronic lung diseases can cause severe, protracted illness and ultimately death. This means that patients suffer long hospitalisation times and healthcare systems incur high costs. Research on lung stem cells helps to understand how tissue is maintained, and how the disruption and failure in mechanisms of repair after injury (for example, caused by smoking or air pollution) contribute to the development of these diseases. Understanding how lung stem cells achieve tissue regeneration is helping to develop new treatments to restore functional lung tissue.
What do we know?
Stem cells are those that are capable of dividing to replace cells that have been lost, either during normal organ turnover or following injury. Identifying these cells within the lung is an important task. In healthy people, lung stem cells maintain the normal lung structure. When they receive specific cues, stem cells divide to replace old or damaged lung cells. In chronic lung diseases, stem cells may be exhausted due to constant repair activity and therefore, this process can go wrong. Faulty repair mechanisms in the lung can lead to fibrosis, where healthy tissue is replaced by scar tissue. This leads to abnormalities in the structure and function of lung tissue, and symptoms such as breathing difficulties and ultimately death.
Understanding the processes by which stem cells in the lung repair damage, and how stem cell behaviour is altered in chronic lung diseases or severe infections, is crucial for finding new treatments.
What are researchers working on?
In the future, it might be possible to develop therapies which promote cell and tissue regeneration. These could increase the volume of functional lung tissue in patients with chronic lung disease. Most lung stem cell research is performed in either model organisms (typically laboratory mice) or human tissue grown in the lab. We can isolate lung stem cells from donated human tissue and grow them either as 2D cell cultures or as 3D ‘organoid’ cultures, or we can get explants from healthy or diseased human lungs and maintain them in the lab as “lung slices” which resemble mini-organs. This method allows researchers to alter the environment that the stem cells experience and to evaluate how to promote their regenerative capacity. Researchers can, for example, compare healthy stem cells with stem cells from patients. These cultures can also be used as drug screening models, and even serve as a basis for patient precision medicine. In these ways, stem cells are contributing to research efforts to cure lung diseases.
Researchers working on genetic diseases are also working on using modern genetic tools to “correct” the DNA changes that cause inherited genetic disorders, such as cystic fibrosis or primary ciliary dyskinesia. It has been possible to edit the gene linked to cystic fibrosis (CFTR). So far, these gene corrections have only been performed on cells growing in the laboratory. However, scientists are actively working on cell therapy and gene therapy approaches to make the same changes in patients’ lungs.
What are the challenges?
Much of our understanding of lung stem cell biology has come from studies using mice as a model system. For example, cell studies of both mice and human samples have revealed many new cell types that are present in the lungs. When the functions of new cell types are not known, using mice is an excellent way for researchers to study them in an intact lung. Mouse and human lungs have similar anatomy and physiology. However, it has become clear that there are also significant differences in cell populations between mouse lungs and human lungs. Differences also exist between mouse and human ageing processes. These limitations mean that it is always important for researchers to confirm findings in model species using human-based studies and patient data.
Researchers can also use human stem cells in cell cultures. However, such studies only recreate some aspects of human lung biology. They lack many of the environmental cues found in intact lungs in a living organism. As such, there are important roles for both approaches in lung stem cell research.
Stem cells in the lung
Our lungs work hard. Someone who lives to 80 years of age will have taken approximately 600 million breaths and experienced a daily airflow of over 10,000 litres. Mammalian lungs are made up of two distinct regions:
1. The conducting airway tubes, including the trachea, bronchi, and bronchioles.
2. The gas exchange regions, or alveolar spaces.
Some stem cells contribute to initial lung development. Others help to repair and regenerate the lung throughout life. In adult lungs, scientists have discovered that the regions of the lung each contain unique types of stem cells located in specific niches. Normally, many stem cells are present throughout each region. These cells divide to replace old or damaged lung cells, which maintains the healthy structure and function of lung tissue.
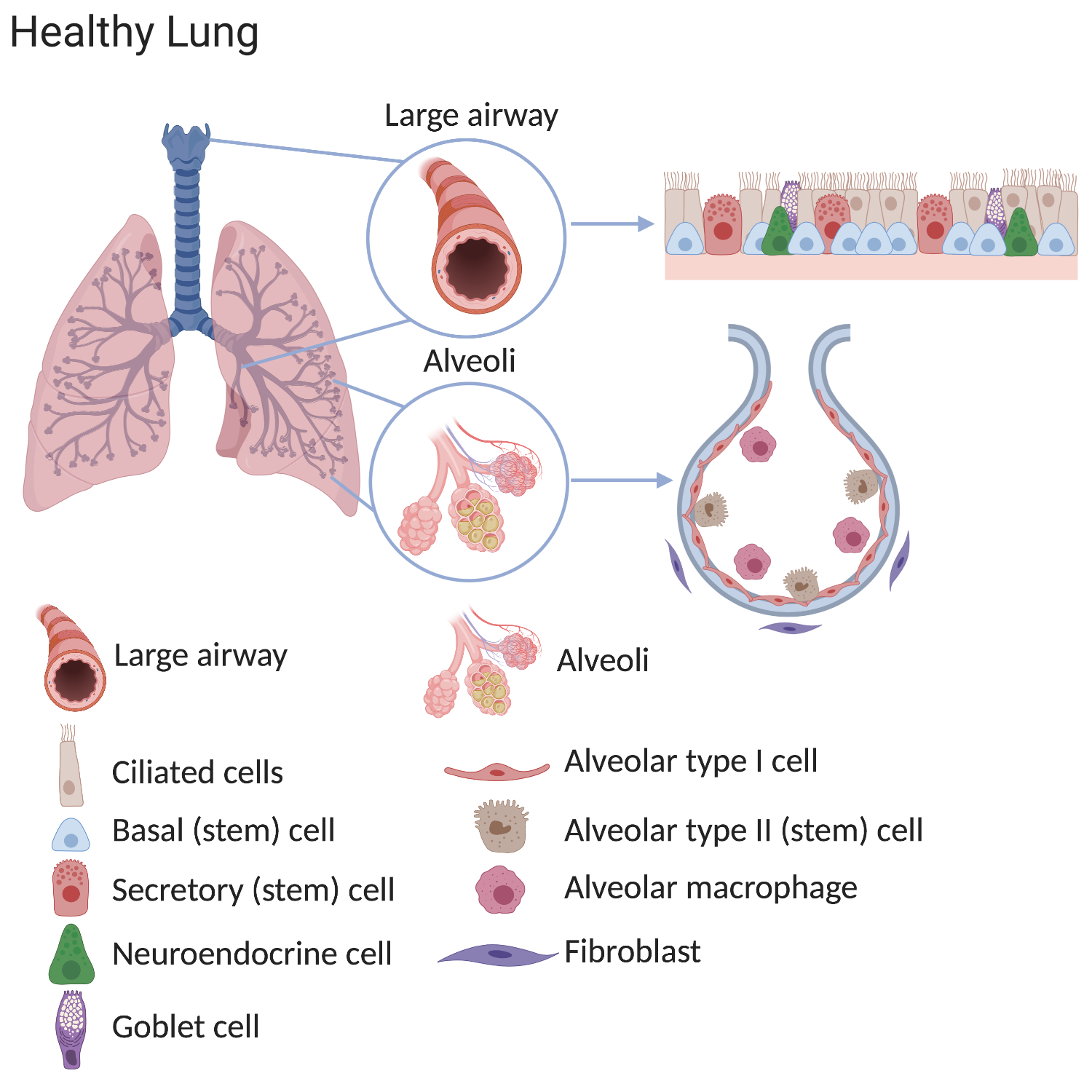
In diseased lungs, the stem cells and the surrounding environment (stem cell niche), can become altered. Consequently, normal lung structure and main functions - filtering the air we breathe and gas exchange - often cannot be well maintained. The causes of these changes are not completely known but can include smoking, air pollution, severe infections and genetic factors.
In the alveoli of COPD lungs there are fewer alveolar type I cells (the cells responsible for gas exchange). In healthy lungs, alveolar type II cells can make type I cells. While this process still happens in COPD lungs, it is less efficient which explains the reduced numbers of alveolar type 1 cells. Importantly, the environment surrounding stem cells also changes in disease. There are more macrophages and fibroblasts found in the alveoli of COPD lungs. These surrounding cells can provide signals which change normal stem cell behaviour.
The stem cell populations in the lung include:
- tracheal basal cells
- bronchiolar secretory cells (known as club cells)
- alveolar type 2 cells
Division of these stem cells is thought to be sufficient to maintain the lung's structure throughout normal adult life. In response to specific type of injuries distinct progenitor cell populations may respond to enable tissue repair, depending on the region and severity of the injury.
Approximately 300 million people currently have asthma, being the most common chronic disease in children affecting 14% of children worldwide.
The lung can roughly be divided into the airways and the alveoli. The air that we breathe is filtered in the airways and oxygen is transferred to the bloodstream in the alveoli.
In a healthy lung, the different types of stem cells slowly produce new lung cells at the same rate as cells are lost in order to maintain a normal lung structure. Lung stem cells can also rapidly respond to lung injury by making new cells more quickly. In lung diseases these maintenance and repair processes are affected, meaning that fewer functional cells are produced than are required. This means that the lung’s ability to supply the body with oxygen is reduced. This can lead to the symptoms we associate with illness, and in extreme cases, to death.Differences in the composition and behaviour of lung cells have been confirmed in various lung conditions. The lungs of patients with chronic lung diseases show alterations in the airways and in the alveoli, especially after exposure to cigarette smoke.
For example, the large airways of patients with COPD show:
- an increased number of basal cells (basal cell hyperplasia)
- loss of ciliated cells
- an increased number of mucous-secreting goblet cells.
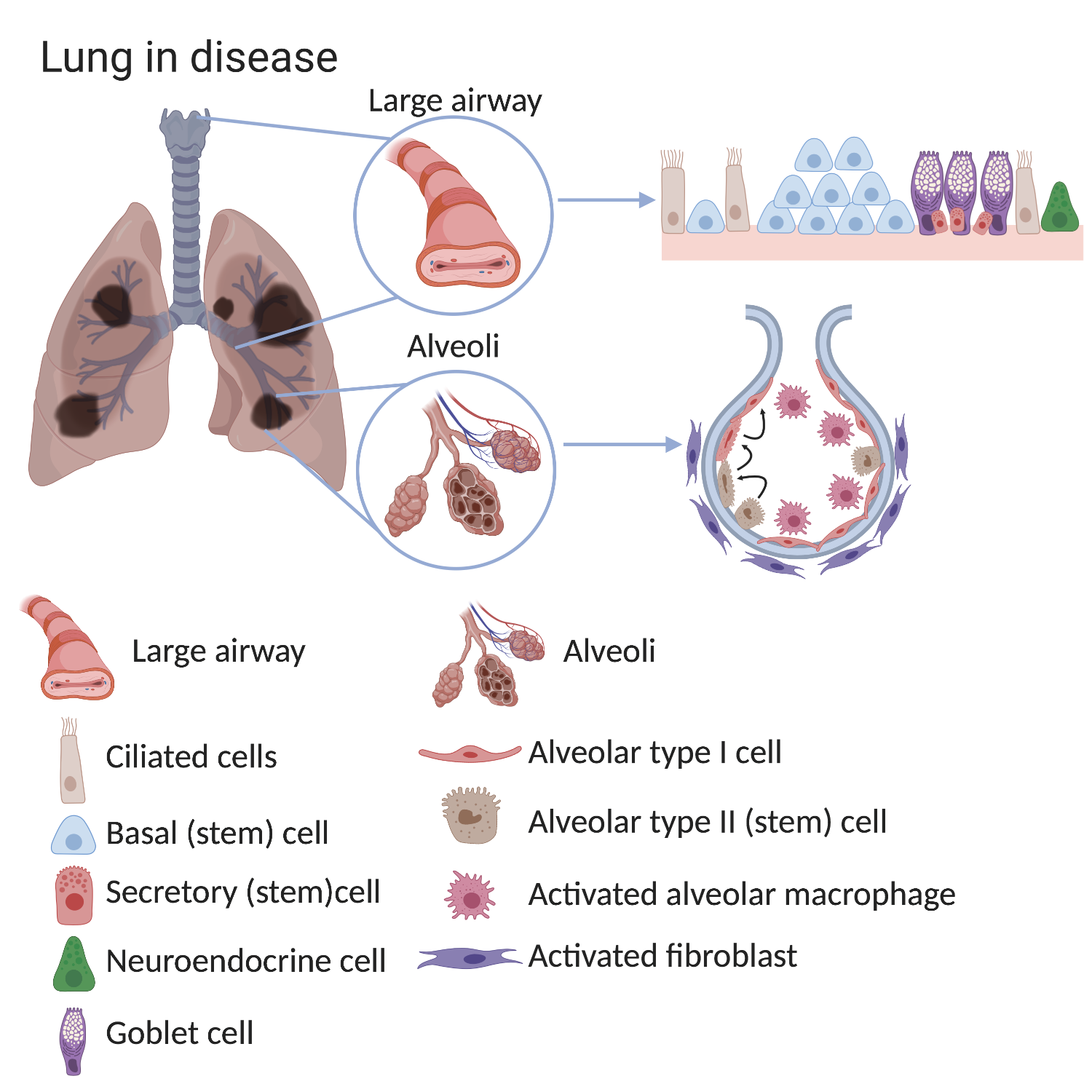
In diseased lungs, the stem cells and the surrounding environment (stem cell niche), can become altered. Consequently, normal lung structure and main functions - filtering the air we breathe and gas exchange - often cannot be well maintained. The causes of these changes are not completely known but can include smoking, air pollution, severe infections and genetic factors.
In the alveoli of COPD lungs there are fewer alveolar type I cells (the cells responsible for gas exchange). In healthy lungs, alveolar type II cells can make type I cells. While this process still happens in COPD lungs, it is less efficient which explains the reduced numbers of alveolar type 1 cells. Importantly, the environment surrounding stem cells also changes in disease. There are more macrophages and fibroblasts found in the alveoli of COPD lungs. These surrounding cells can provide signals which change normal stem cell behaviour.
How might gene and cell therapy help?
250 million people suffer chronic obstructive pulmonary disease (COPD) and 3 million die from it every year, being the third cause of death worldwide.
Several lung diseases are caused by alterations in one particular gene, including cystic fibrosis, primary ciliary dyskinesia and congenital alveolar proteinosis.
As an example, cystic fibrosis (CF) is caused by changes in the gene that encodes the cystic fibrosis transmembrane conductance regulator (CFTR). This protein is important for regulating the balance of water and salts in tissues. When the protein doesn’t function properly there is a build-up of thick mucus in the lungs, which impairs their function and makes patients susceptible to infection. The goal of gene therapy to treat diseases like CF by restoring the normal function of the affected protein by modifying the DNA in lung cells. Since lung cells are continuously renewing, it might be possible to avoid the need for repeated doses of gene therapy by targeting lung stem cells.
Gene therapy and lung disease
Gene therapy involves transferring genetic sequences into cells. This is challenging as DNA does not readily pass through cell membranes. Gene therapy therefore requires a vector to transport the genetic material into the cell.
Replication-deficient viruses (those which cannot multiply within cells and infect other surrounding cells) are used as vectors, as these can carry DNA into cells. The delivery of these viral vectors directly to the lungs might allow DNA modification within patients. This could involve:
- the addition of a functional copy of the gene to the genome
- gene editing (where the patients’ dysfunctional gene is corrected).
An alternative approach is to combine cell and gene therapies. We can grow large numbers (expand) of lung stem cells from human lungs in the laboratory. It is therefore possible to grow cells from patient biopsies, infect them with a virus to allow expression of healthy CFTR and then return these corrected cells as a transplant. Current methods to expand cells could, theoretically allow for this to be done on an individual, ‘per-patient’ basis. This would avoid problems associated with the rejection of donor tissue. Most challenging, though, is establishing how to deliver these cells to the injured site, and whether they will be able to integrate into the tissue (engraft) and regenerate the tissue.
Current research
Scientists working in the field of gene therapy have investigated the use of multiple types of viruses (including adenoviruses, retroviruses and lentiviruses) to transfer DNA to cells. Researchers are also investigating other non-viral gene transfer approaches, including both biological and inorganic approaches. These would avoid the use of viruses, and potentially allow the transfer of much larger DNA sequences to the target cells. A lot of research also focuses on the best way to deliver these vectors to the cells; for example, whether this might be possible through non-invasive aerosol delivery in lung disease
In terms of cell therapy, great strides have been made in terms of both:
- our ability to expand lung stem cells for a long time in cell culture
- our ability to perform genome modification of these cells in a manner that might eventually prove therapeutic.
In comparison to the skin, where transplantation of skin grafts has been possible for several decades, an important outstanding question in the lung field is how cultured (and gene-corrected) cells might be efficiently transplanted within the lungs. Furthermore, more work is required to ensure that these cells would be capable of maintaining lung tissue for the lifetime of the patient.
Many open questions remain when it comes to transplanting laboratory-grown cells and tissue. How many cells are required, and what is the best route of administration? Will transplanted cells engraft and survive? Will these cells behave ‘normally’ after transplant? Will they be able to regenerate the tissue in the setting of a damaged environment?
In addition to the administration of exogenous stem cells (cells transplanted into the body), the stimulation of endogenous progenitor cells (those located within the body) has been also proposed as a therapeutic approach to promote lung regeneration. This approach faces other challenges. It is unknown whether endogenous stem cells in diseased lungs are able to accomplish successful lung regeneration: those cells may be already damaged, and the environment is also altered.
Next steps
91% of the world population lives in places where air quality exceeds the limits suggested by the WHO. This significantly influences the development or worsen of chronic obstructive pulmonary disease (COPD), asthma, interstitial pulmonary disease, pulmonary hypertension or acute respiratory infections
As scientists improve their knowledge of how human lung stem cells contribute to lung development, maintenance and regeneration our ability to direct these processes with new therapeutics will improve.
The development of new models is particularly necessary. This will allow scientists to investigate the mechanisms involved in repair and regeneration, and to find out how to promote lung regeneration to treat human lung disease. Novel animal models and ex vivo platforms can provide a basis for a deeper understanding of lung physiology and pathology in humans, and allow the investigation and design of more curative therapies.
Clinical trials are currently being conducted for treatment of cystic fibrosis. These trials aim to overcome some of the challenges of delivering a functional CFTR gene to patient (stem) cells.
However, there are still significant hurdles to overcome before cell and gene therapies are routinely used within the context of respiratory disease. More pre-clinical research is still required. However, significant progress is being made in terms of our ability to safely edit the DNA sequence of lung stem cells. This provides hope that this approach might prove a viable route for treating respiratory genetic diseases in the long term.
Research is still needed to develop strategies for using cell therapies in lungs already damaged by disease. Scientists are investigating the potential of these therapies, in combination with other approaches, to restore damaged structures, and regenerate tissue to improve patients’ breathing once more.in combination with other therapeutic approaches targeting pathological events and addressed towards restoring lung architecture and regenerate functional alveolar units for breathing.